A crack in the cellular energy dam
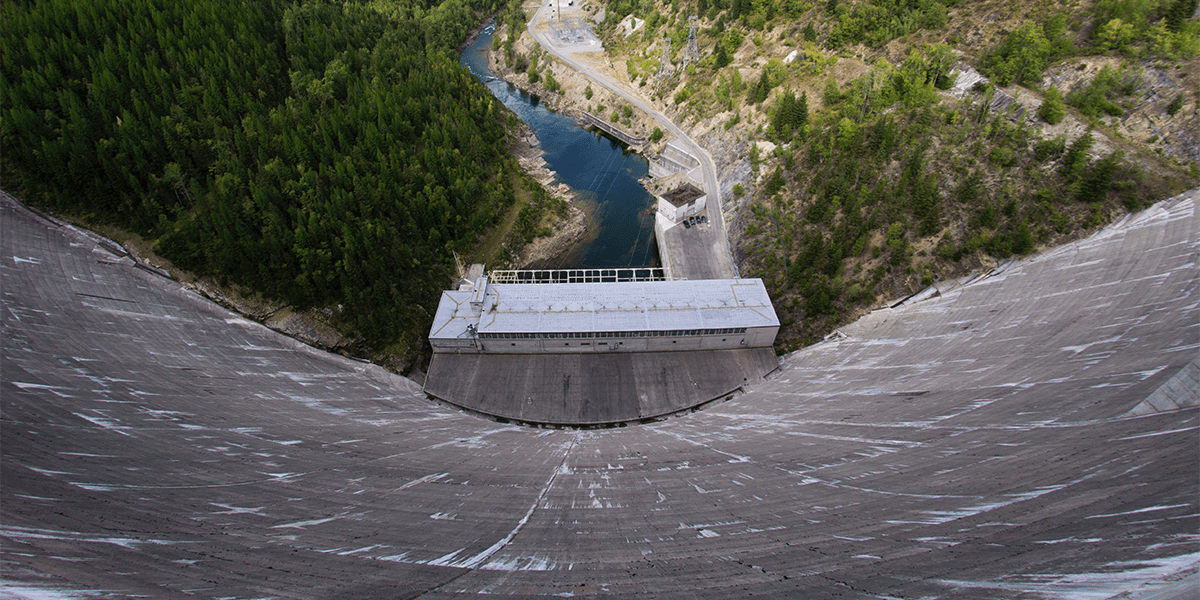
Each day, the Hoover Powerplant produces enough energy to power one house for over a thousand years1. Such immense amounts of power are generated from the controlled flow of water through turbines at the base of the Hoover Dam. It makes sense that water rushing through a spinning gate can create energy. But what would you think if someone told you that the dam might be more efficient with a crack in it? It’s probably safe to say that you’d have a few questions. So did scientists when they found that a version of the UCP2 gene—a gene that’s capable of decreasing energy production in humans—may help our body respond to exercise.
During exercise, it’s not just our muscles that need energy—we need it to breathe oxygen into our lungs, to pump blood through our veins, and to power our motivational thoughts that get us to the gym in the first place (among many other things). In order to fulfill such a demand, almost every cell in our body has built-in powerhouses known as mitochondria.
Mitochondria are bacteria-like structures that produce the vast majority of energy our body uses. To do so, they have an internal membrane that acts like a dam. On one side of the membrane is a “lake” of hydrogen ions, which contrasts with the relative lack of hydrogen on the other side. In this membrane, there are special proteins that allow a controlled amount of hydrogen to flow through the membrane to the other side. Like water flowing through turbines at a dam, the flow of hydrogen produces a force that the mitochondria capture and turn into energy.
Hydrogen produces a force that the mitochondria capture
To be sure, it’s a complex process. But it’s also beautifully intuitive—the principles governing energy production in our cells are the same as those at the Hoover Dam. Or so we thought.
The UCP2 gene is a member of the uncoupling protein gene family. These genes code for proteins that disrupt the energy production process. Like a crack in a dam, uncoupling proteins are inserted in the mitochondrial membrane where they allow hydrogen to flow down their concentration gradient without having their energy captured. There is still energy that’s released as the hydrogen flows, but without the right machinery there to capture it, the energy is released as heat. Researchers have found that this is one way our bodies keep warm in times of need—fat cells can use uncoupling proteins to generate heat2,3.
So why would a protein that decreases our body’s ability to capture energy be helpful during exercise? At the moment, scientists can’t answer this question completely, but they do have some evidence that helps lead them to a possible answer.
During the process of energy production, mitochondria may incidentally produce ROS—oxygen molecules that are highly unstable and capable of damaging parts of the cell. We have many defense mechanisms in place to protect against the harmful effects of ROS, but when we exercise, the amount of ROS increases throughout the body3. Researchers have found that some cells in our body will increase UCP2 gene activity, resulting in more UCP2 protein being made during exercise. Based on studies in mice, it’s believed that this increase in UCP2 helps reduce the amount of ROS and potentially protects us from ROS damage2,3.
UCP2 may protect us from ROS
Curiously, genetic studies have also found that, when compared to non-athletes, elite endurance athletes are more likely to have a specific variant—a heritable change in the DNA sequence—in the UCP2 gene4-8. Researchers are still studying this connection in an effort to explain why this association is there, and what effect this variant may have on UCP2 function. Areas of interest include studying how the UCP2 gene affects energy use and ROS production during exercise. Thus far, research into UCP2 and other genes associated with athleticism has shown us that a person’s athletic ability is the result of their environment, their training, and their genetics. This means that simply having the UCP2 variant that’s associated with endurance athleticism isn’t enough to make someone an elite athlete; similarly, not having the variant doesn’t mean you’ll never finish a marathon. It’s just one factor among many.
While we may not yet know why there is a link, it does seem to be the case that the endurance-associated variant in UCP2 is more common in people who excel at endurance sports4-8.
- Bureau of Reclamation, and Lower Colorado Region Web Team. “HOOVER DAM.” Shasta Dam – Northern California Area Office | Bureau of Reclamation, www.usbr.gov/lc/hooverdam/faqs/powerfaq.html.
- Rousset, S., et al. “The Biology of Mitochondrial Uncoupling Proteins.” Diabetes, vol. 53, no. Supplement 1, 2004, doi:10.2337/diabetes.53.2007.s130.
- Azzu, Vian, and Martin D. Brand. “The On/off Switches of the Mitochondrial Uncoupling Proteins.” Trends in biochemical sciences 35.5 (2010): 298–307. PMC. Web. 18 Sept. 2018.
- De Souza, Bianca M. et al. “Associations between UCP1 -3826A/G, UCP2 -866G/A, Ala55Val and Ins/Del, and UCP3 -55C/T Polymorphisms and Susceptibility to Type 2 Diabetes Mellitus: Case-Control Study and Meta-Analysis.” Ed. Qingyang Huang. PLoS ONE 8.1 (2013): e54259. PMC. Web. 18 Sept. 2018.
- Gamboa, Ricardo et al. “The UCP2 -866G/A, Ala55Val and UCP3 -55C/T Polymorphisms Are Associated with Premature Coronary Artery Disease and Cardiovascular Risk Factors in Mexican Population.” Genetics and Molecular Biology 41.2 (2018): 371–378. PMC. Web. 19 Sept. 2018.
- Ahmetov, Ildus I., and Olga N. Fedotovskaya. “Current Progress in Sports Genomics.” Advances in Clinical Chemistry, 11 Apr. 2015, pp. 247–314., doi:10.1016/bs.acc.2015.03.003. Web. 19 Sep. 2017
- Ahmetov, Ildus I., et al. “The Combined Impact of Metabolic Gene Polymorphisms on Elite Endurance Athlete Status and Related Phenotypes.” Human Genetics, vol. 126, no. 6, Apr. 2009, pp. 751–761., doi:10.1007/s00439-009-0728-4.
- Sessa, Francesco, et al. “Gene Polymorphisms and Sport Attitude in Italian Athletes.” Genetic Testing and Molecular Biomarkers, vol. 15, no. 4, 2011, pp. 285–290., doi:10.1089/gtmb.2010.0179.
Categories