DNA Considerations for Space Travel and Colonization
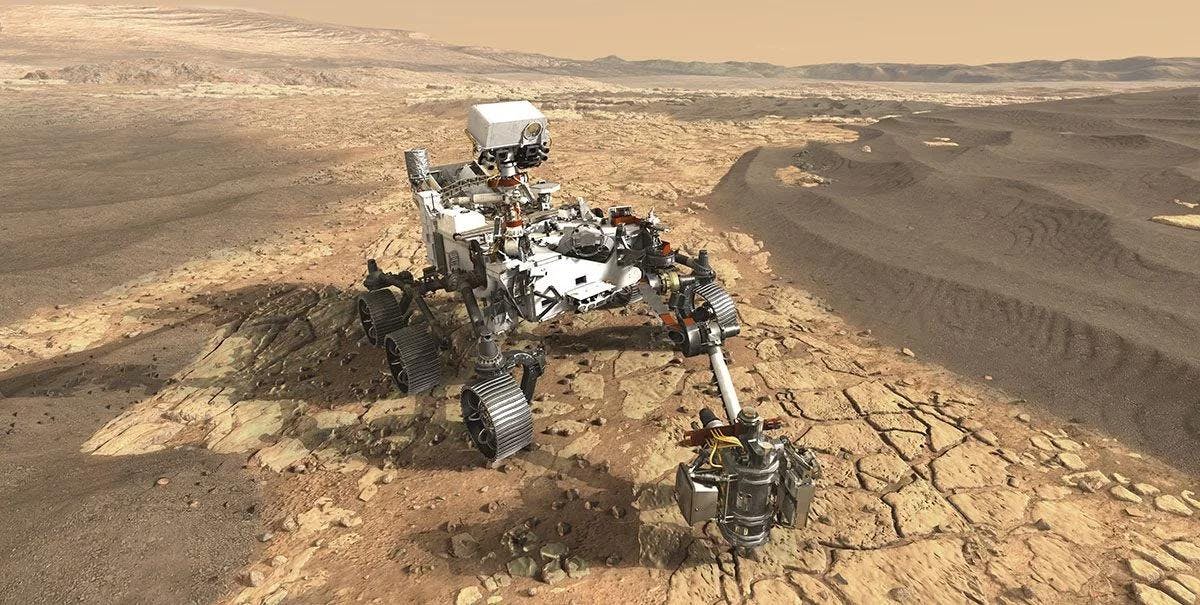
Can humans evolve to make space travel safer?
Near the cement-sealed Chernobyl nuclear power plant, site of the worst nuclear accident in history, you might expect a wasteland—a stretch of Ukrainian countryside completely uninhabitable by organisms of any kind.
But you’d be wrong. Wolves hunt their prey. Owls soar overhead. Somehow, nature is persevering.
How this is happening isn’t entirely clear, because we know that radiation is a significant mutagen that can have adverse effects on life forms. In April of 1986, Chernobyl released immense amounts of radiation into the surrounding environment. Though humans still don’t live there due to the dangers of radioactivity, wildlife has remained in the region, which has prompted scientists to ask if these animals are evolving to withstand the high amounts of radiation 1.
The phenomenon isn’t just interesting here on Earth—it’s also interesting for the possible implications it has on space travel. Besides emitting light, stars bathe the universe in particle radiation with periodic bursts from solar explosions 2. Humans have big ambitions to go to Mars and beyond. Like the wildlife surrounding Chernobyl, humans will have to learn how to live in a radioactive environment if we want to go to Mars. So, as we venture into the new frontier, how will we stay safe? Could our DNA evolve to help us?
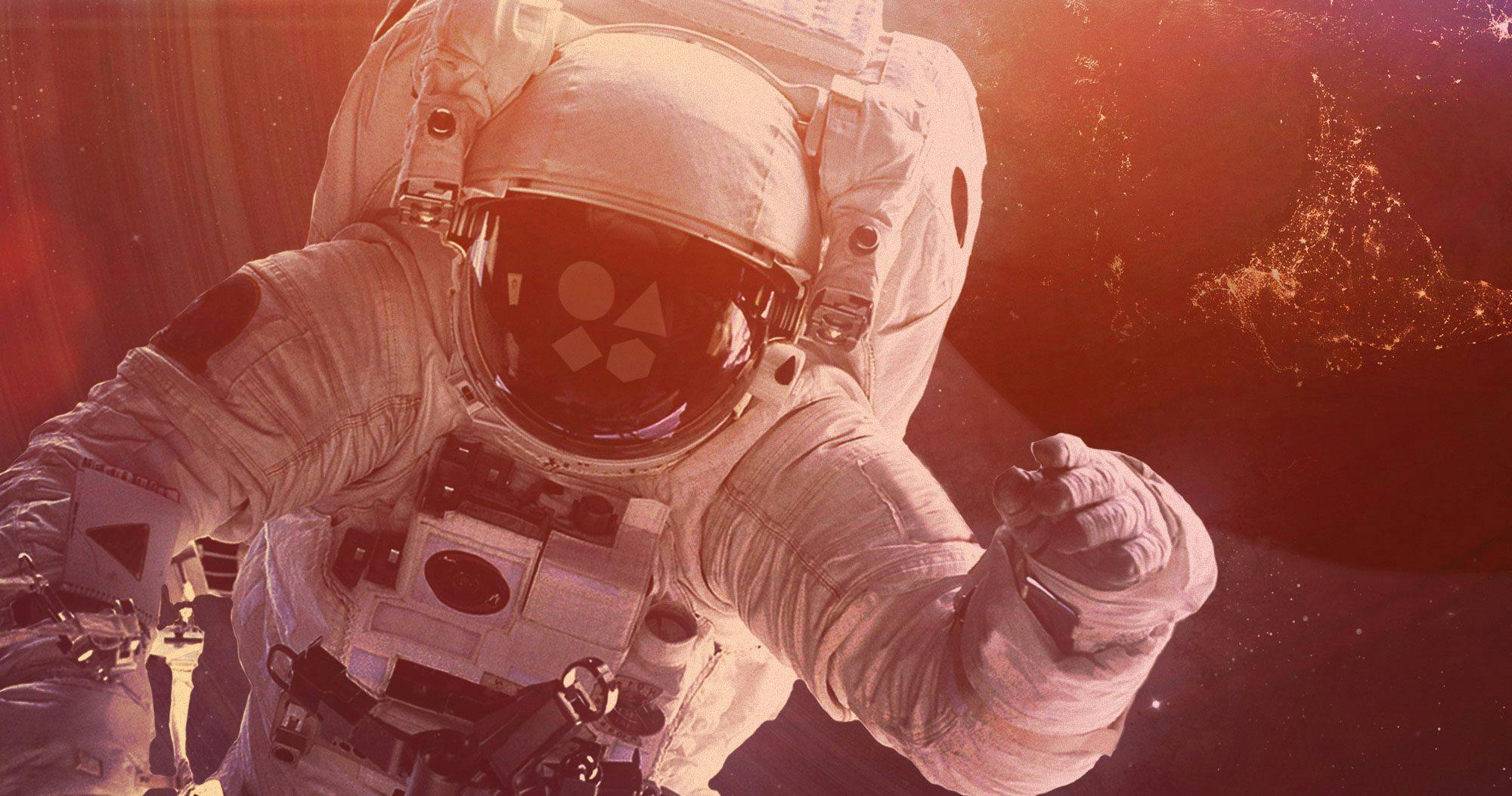
DNA is one of the big reasons that radiation is dangerous for us. When assembled into the right order, DNA is like an instruction manual for how to build life. Naturally, it’s important to try and protect the DNA and prevent it from being damaged. Just like ripping pages out of a book, it would be bad for a cell to have its DNA broken—which is exactly what radiation can do. Radioactive particles penetrate into a cell and collide into the DNA and other molecules with large amounts of energy. This powerful collision breaks chemical bonds and can cause multiple types of DNA damage. Luckily, most organisms have evolved elaborate mechanisms to repair damaged DNA. Depending on the type of damage, a cell can rewrite the portions of DNA that were broken, or fix it in other ways that are somewhat similar to taping a torn page in a book.
These repair mechanisms are extremely effective, but they can sometimes lead to mutations. In the book analogy, this would be like rewriting a torn page and accidentally adding in a typo. Mutations may be harmless, or they could cause a cell to behave erratically and lead to disease. For this reason, when there is too much damage in a cell, they will trigger a safety measure that causes the cell to die and prevent potential disease development.
Studies of plants and animals in the Chernobyl region have shown significant increases in the amount of DNA damage in some animals, and there are many indications of a higher mutation rate among them 1,3,4. Yet, they’re still able to survive. It’s been suggested that they’ve begun to evolve more efficient mechanisms for coping with the DNA damage, but research is ongoing.
Elsewhere, there are examples where organisms have evolved an incredible ability to survive radiation induced DNA damage. The bacteria Deinococcus radiodurans is famously able to survive extreme levels of radiation without dying, and is even able to preserve its DNA sequence relatively well 5,6. How it does this is not entirely clear, because it has many of the same DNA repair genes as humans do. But scientists are very interested in learning more about how this bacteria has adapted to live under such extreme conditions. Understanding how an organism survives radiation could influence how we approach radiation protection for ourselves.
So it appears that life can flourish under high levels of radiation, but it requires a coping mechanism to allow survival despite DNA damage and mutation. For the most part, radiation from space isn’t a problem for us because the earth is surrounded by a magnetic field that prevents the radioactive particles from reaching us 2. However, such a field is not present around Mars, and it’s likely that any human colony living there will be exposed to continuous radiation at rates much higher than here on Earth.
Astronauts have been going to space for decades but have mostly remained within the magnetosphere. By staying within its protection—and by limiting the length of time astronauts spend in space—these temporary space travelers have been shielded from the more extreme radiation exposure that would be experienced on a longer (or permanent) trip, like heading to Mars. Ongoing studies aim to determine if our body is capable of repairing DNA damage incurred during space travel and how our body may or may not cope with it 2. Studies in which astronaut Scott Kelly went to space for nearly a year aboard the ISS while his twin brother remained on Earth as a control provided evidence that the human body may increase expression of DNA damage repair genes to help protect our DNA in space, but further studies are needed to both confirm these results and understand their implications.
So, all of this brings us back to the main question: will our DNA evolve and come to our rescue as we establish homes on Mars? Life is always evolving, so there’s no question that humans will continue to do so. But it’s not clear yet whether the amount of radiation in space could affect us in such a way as to influence our evolution. Additionally, evolution takes place on a very large time scale, so it would take thousands of years for this to happen (at minimum). That’s not to say we won’t get to Mars before that—but to do it safely, we’ll still need technology to shield us from the harsh effects of the final frontier.
The Founder Effect
Imagine for a moment that 20 people were sent from earth to establish a human colony on Mars. From these people, a new society would have to be formed. But what kind of society would it be? What language would the people speak, and what would they look like? From this short thought experiment, you can see that isolating a small batch of people from a larger population can have a dramatic effect on their society—some cultural and physical traits will be overrepresented, while others may even disappear.
While this thought experiment may seem to be nothing more than science fiction, it actually demonstrates a concept that has molded populations throughout history. This concept is known as the founder effect.
The founder effect is when a small population of organisms are separated from the larger population, causing some traits to be disproportionately represented relative to the larger population. To understand concepts like this, visualizations can be helpful, so check out the figure below. In this example, you can see how the separation of one group leads to a population that is quite different from the original population that they came from. This concept is important because it helps describe how some populations of humans have developed unique traits—and why some groups have increased rates of certain diseases relative to others.
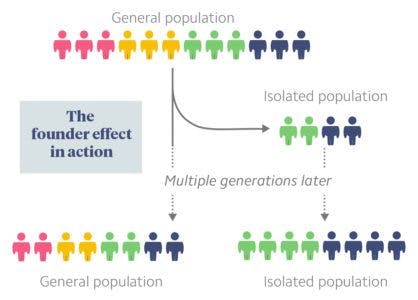
Iceland is a good example of how the founder effect can change a population. Approximately 1,100 years ago, people emigrated from Scandinavia, Scotland, and Ireland to colonize the island of Iceland 7,8. Since that initial wave, there has been very little immigration to the island, which means their population of nearly 320,000 people are mostly descended from a small group of ancestors 9. It is theorized that some people among these early ancestors carried a variant in the BRCA2 gene (for you geneticists, it’s c.771_775del5)9 that has been passed on and become relatively more common in Iceland than many other populations, which means a higher percentage of Icelanders may be at an increased risk of developing breast and ovarian cancer. In this case, about 1 out of 250 Icelanders is a carrier of this BRCA2 variant, whereas this variant is less common around the world 9,10.
Founder Effect vs. Bottleneck
The founder effect is very similar to a population bottleneck. In both cases, a group of people from a larger population becomes isolated, but the difference lies in the reason for this isolation.
A bottleneck occurs when there is some catastrophe leading to a large die-off within a population (or at least a large drop in the reproductive pool), such as what happens to the non-resistant bacteria in this video about antibiotic resistance (the survivors make it through the bottleneck).
The founder effect is defined by a group of people becoming isolated, geographically or culturally, and remaining isolated for a period of time.
Increases in rare diseases following the isolation of a group of people have been well studied. A notable example is that of the Fugate family, more commonly known as the “Blue People of Kentucky.” In the early 1800s, a young man moved to an isolated village in Kentucky. This man, Martin Fugate, carried one copy of a rare autosomal recessive variant (meaning the full effects of this variant are not seen unless you have two copies of it, one from each parent) in the CYP5R3 gene 11. This variant causes a person to have abnormally high levels of methemoglobin, a protein similar to hemoglobin but that is unable to carry oxygen. Too much of this protein can result in low levels of blood and tissue oxygenation, which leads to a bluish hue in the skin—hence the common term for the condition, “blue skin disease.”
Martin Fugate was a carrier of the CYP5R3 variant, and coincidentally, so was his wife Elizabeth Smith 9. Their community remained isolated for multiple generations. This caused many people in the Fugate family to inherit this variant in the CYP5R3 gene, resulting in high levels of methemoglobin and bluish skin. Once the family dispersed from their isolated community, the rate of blue skin disease decreased significantly 11. Here the founder effect is apparent: Martin Fugate and his wife were founders. Because they both carried a rare disease variant, and because the family remained isolated, the disease became far more prevalent in their community than it is in the general population 11.
There are many examples of the founder effect, most of which focus on how it has lead to increased disease rates in certain populations. This is because there is a significant medical benefit to society from understanding how diseases are inherited and what genes are involved. However, the founder effect does not only affect disease rates. In the same way that a rare disease can be overrepresented in an isolated population, so too can rare traits such as hair color or skin color. For example, in the Solomon Islands, there is a unique genetic variant affecting hair color that leads to blonde hair in much greater proportions than elsewhere 12.
Isolation of communities can have a profound effect on their culture by limiting their diversity. In terms of genetics, increased genetic diversity helps to prevent rare diseases from becoming common ones. Human history is rich with isolation events, though, which means the founder effect has helped shape the development of some modern cultures.
So what does this mean for establishing a colony on Mars? There will be many hurdles to overcome when voyaging off our planet—radiation exposure, growing food in a sustainable way, the list goes on. And in the long term, these explorers will need to contend with the founder effect, too, which means they’ll need to be resourceful, resilient, and diverse. Is humankind up for the challenge? We think so!
Citations
- Møller, Anders Pape, and Timothy A. Mousseau. “Strong Effects of Ionizing Radiation from Chernobyl on Mutation Rates.” Scientific Reports 5 (2015): 8363. PMC. Web. 1 May 2018.
- Moreno-Villanueva, María et al. “Interplay of Space Radiation and Microgravity in DNA Damage and DNA Damage Response.” NPJ Microgravity 3 (2017): 14. PMC. Web. 1 May 2018.
- Baker, Robert J. et al. “Elevated Mitochondrial Genome Variation after 50 Generations of Radiation Exposure in a Wild Rodent.” Evolutionary Applications 10.8 (2017): 784–791. PMC. Web. 2 May 2018.
- Ragon, Marie et al. “Sunlight-Exposed Biofilm Microbial Communities Are Naturally Resistant to Chernobyl Ionizing-Radiation Levels.” Ed. Vishnu Chaturvedi. PLoS ONE 6.7 (2011): e21764. PMC. Web. 2 May 2018.
- Makarova, Kira S. et al. “Genome of the Extremely Radiation-Resistant Bacterium Deinococcus Radiodurans Viewed from the Perspective of Comparative Genomics.” Microbiology and Molecular Biology Reviews 65.1 (2001): 44–79. PMC. Web. 2 May 2018.
- Krisko, Anita, and Miroslav Radman. “Biology of Extreme Radiation Resistance: The Way of Deinococcus Radiodurans.” Cold Spring Harbor Perspectives in Biology 5.7 (2013): a012765. PMC. Web. 2 May 2018.
- Helgason, Agnar et al. “Sequences From First Settlers Reveal Rapid Evolution in Icelandic mtDNA Pool.” Ed. Gil McVean. PLoS Genetics 5.1 (2009): e1000343. PMC. Web. 12 Jan. 2018.
- “Letters from Iceland.” Nature Genetics, 2015, doi:10.1038/ng.3277.
- Price, Alkes L. et al. “The Impact of Divergence Time on the Nature of Population Structure: An Example from Iceland.” Ed. Gil McVean.PLoS Genetics 5.6 (2009): e1000505. PMC. Web. 12 Jan. 2018.
- Janavičius, Ramūnas. “Founder BRCA1/2 Mutations in the Europe: Implications for Hereditary Breast-Ovarian Cancer Prevention and Control.” The EPMA Journal 1.3 (2010): 397–412. PMC. Web. 12 Jan. 2018.
- “Finding The Famous Painting of the Blue People of Kentucky.” DNA Science Blog, PLoS, 22 Sept. 2016, blogs.plos.org/dnascience/2016/09/22/finding-the-famous-painting-of-the-blue-people-of-kentucky/.
- Sturm, Richard A, and David L Duffy. “Human Pigmentation Genes under Environmental Selection.” Genome Biology 13.9 (2012): 248. PMC. Web. 12 Jan. 2018.