This gene gives fingers to humans—and legs to snakes
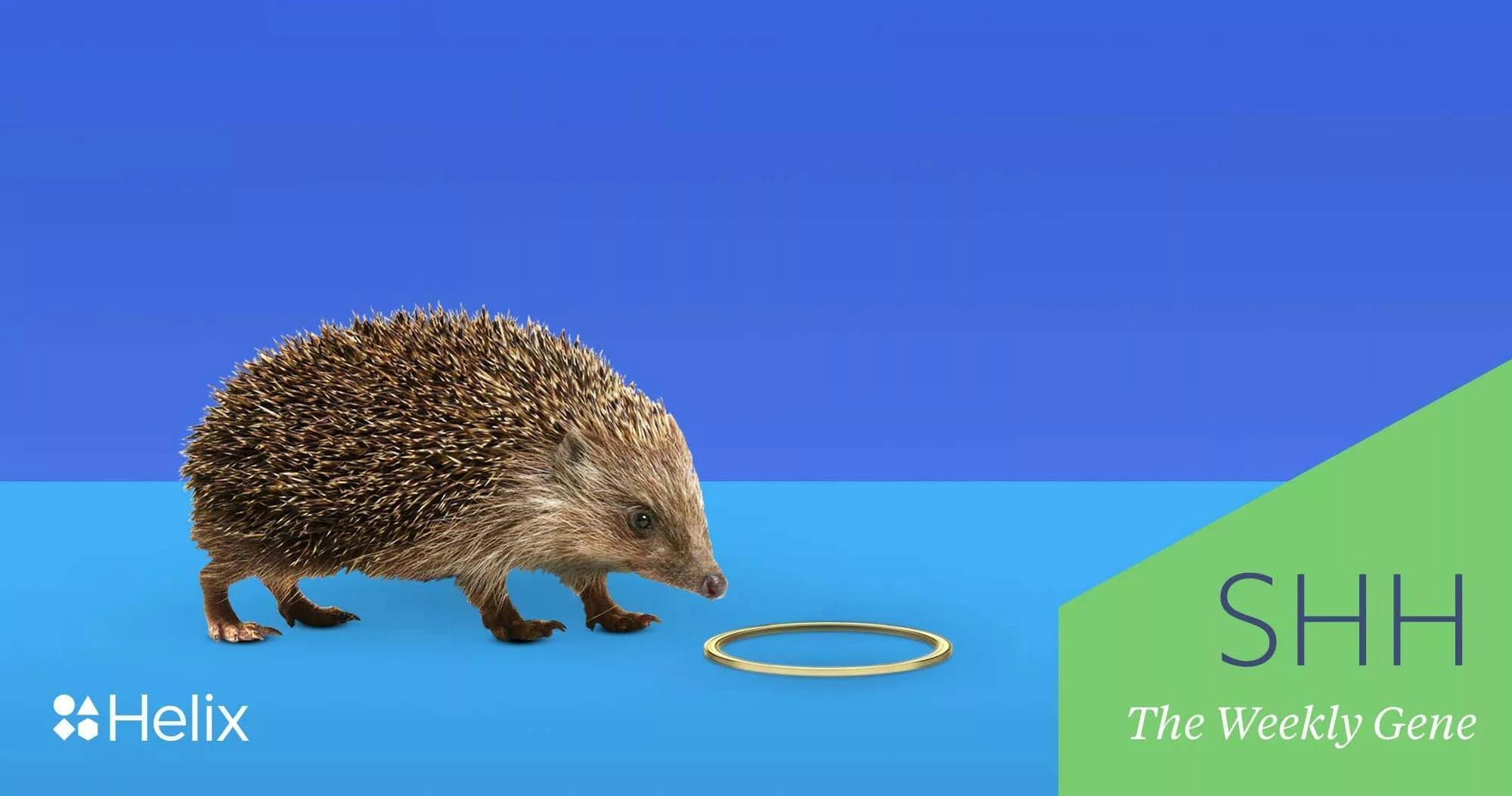
It’s not often that you get to read about walking snakes, Sonic the Hedgehog, and human genetics in the same day. Believe it or not, these very different things are all joined by a fascinating story that’s buried within your genome—the story of human development.
From just a tiny egg, humans develop into multifaceted beings. That single egg will give rise to some very different structures (muscles, eyes, hands, and so on). How this process takes place is still an area of active research, but what we do know is that it’s incredibly complex, involving a web of genes that turn on and off in a specific order, in specific locations, at specific times. Within this gene network lies a series of master regulators that influence many processes of development. One such master gene is known as the Sonic Hedgehog gene.
Gaming genetics
The SHH gene really is named after the video game character! Scientists studying genetics with fruit flies had already discovered a related gene and named it hedgehog because mutations in it caused fly embryos to resemble hedgehogs. When scientists discovered the related vertebrate version of the gene, they were inspired to name it after the video game character thanks to a magazine sporting the blue figure on its cover7.
In the video game world, Sonic the Hedgehog is a fast-moving blue creature with spikes known for his ability to run and jump. In the genetics world, Sonic Hedgehog is a gene that helps orchestrate the proper development of numerous diverse body parts including the limbs we use for running (and the brains we use to play the video game)1,2. The Sonic Hedgehog gene produces a protein of the same name, usually shortened to just SHH, which helps cells talk to one another. The protein is released from a cell and diffuses to neighboring cells where it interacts with special SHH-receiving proteins. This interaction triggers a chain reaction within the receiving cell, and ultimately stimulates the cell to turn specific genes either on or off (many of which help give a cell its identity). By controlling these important genes, SHH acts as a master regulator during development.
How often do you contemplate the difference between your thumb and your pinky finger? If you’re like me, it’s only when trying to fandangle a keyboard short-cut that requires some fancy finger work. But chances are you don’t go so far to dissect how the cells of one part of your hand could differentiate their roles and form their correct digits. Well, let us take the mental burden off of you and give you the answer (or at least part of it): SHH
Very early on in development, when the embryo is just an elongated mass of cells, one group of cells begins to bulge out from the mass. This group will continue to bulge outwards to eventually form a limb (it could be an arm or a leg). During this process, the SHH gene is turned on in the bottommost part of the bulge. The localized production of the SHH protein causes a gradient in the bulge such that the lower part has high amounts of SHH, and the upper part has low amounts. Your fingers actually get their identity based on the amount of SHH they’re exposed to in these early stages: Your pinky was bathed in high amounts of SHH, while your thumb had none2-4. Small mutations that affect SHH signaling can cause a person to have differing numbers of digits (fingers or toes). For example, variants that cause no SHH to be produced can result in the near complete loss of digits, while too much SHH can cause a person to have extra fingers with atypical identities4.
So what does this have to do with walking snakes? Interestingly, changes in the DNA that affect the SHH gene may explain why snakes don’t have legs—anymore. Fossils of ancient snakes show that, at one point in time, snakes had legs5,6. They then lost those these limbs through evolution, gained them back again, and lost them once more. In fact, some modern day snakes still have structures that resemble rudimentary legs. During embryonic development, Boa constrictors develop a femur-like bone and cartilage in the form of a tibia, fibula, and foot. Before the egg hatches, though, these structures are destroyed6. Research suggests that this is due to the early production of SHH protein which helps form these initial structures. Early shut-off of the SHH protein leads to incomplete signaling, and loss of the developing limb6.
At the genetic level, there’s a region of DNA found in nearly all vertebrate animals, from fish to humans. This region helps control when and to what extent the SHH gene is used2,3,5. Scientists found that some snakes have variations in this DNA sequence that prevent the extended production of SHH during limb development. More advanced snakes (like cobras) have several variations in this region that totally prevent the production of SHH protein and prevents even the early formation of legs6. Researchers have hypothesized that snakes developed and lost legs repeatedly throughout history due to various changes in the DNA regulating SHH6. This is still a topic of ongoing research, but it’s fascinating (or terrifying!) to think that snakes are just a few DNA variations away from being able to walk.
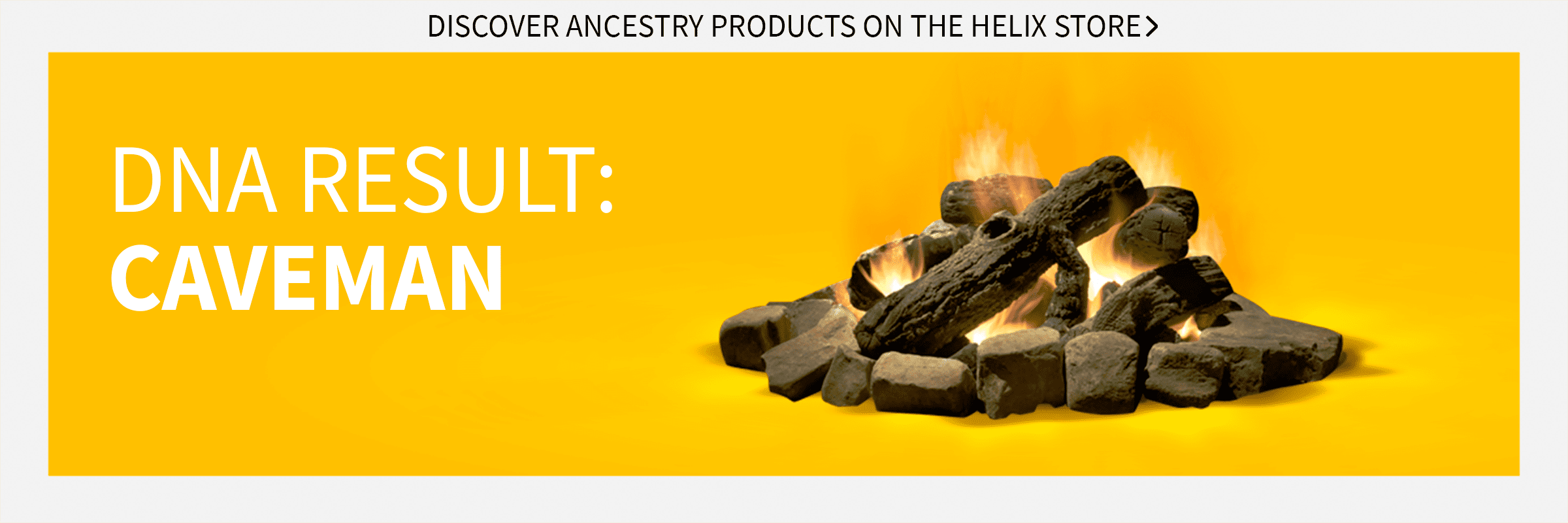
1Dessaud, E., et al. “Pattern formation in the vertebrate neural tube: a sonic hedgehog morphogen-Regulated transcriptional network.” Development, vol. 135, no. 15, 2008, pp. 2489–2503., doi:10.1242/dev.009324. Web. 6 Mar. 2018.
2Sheeba, Caroline J., et al. “Getting a handle on embryo limb development: Molecular interactions driving limb outgrowth and patterning.” Seminars in Cell & Developmental Biology, vol. 49, 2016, pp. 92–101., doi:10.1016/j.semcdb.2015.01.007. Web. 6 Mar. 2018.
3Lettice, Laura A., et al. “The Conserved Sonic Hedgehog Limb Enhancer Consists of Discrete Functional Elements that Regulate Precise Spatial Expression.” Cell Reports, vol. 20, no. 6, 2017, pp. 1396–1408., doi:10.1016/j.celrep.2017.07.037. Web. 6 Mar. 2018.
4Anderson, Eve, et al. “Human limb abnormalities caused by disruption of hedgehog signaling.” Trends in Genetics, vol. 28, no. 8, 2012, pp. 364–373., doi:10.1016/j.tig.2012.03.012. Web. 6 Mar. 2018.
5Abbasi, Amir Ali. “Evolution of vertebrate appendicular structures: Insight from genetic and palaeontological data.” Developmental Dynamics, vol. 240, no. 5, 2011, pp. 1005–1016., doi:10.1002/dvdy.22572. Web. 6 Mar. 2018.
6Leal, Francisca, and Martin J. Cohn. “Developmental, genetic, and genomic insights into the evolutionary loss of limbs in snakes.” Genesis, vol. 56, no. 1, Feb. 2017, doi:10.1002/dvg.23077. Web. 6 Mar. 2018.
7Mossman, Kaspar. “Profile of Clifford Tabin.” Proceedings of the National Academy of Sciences of the United States of America 106.21 (2009): 8407–8409. PMC. Web. 6 Mar. 2018.