How does DNA let you grab oxygen?
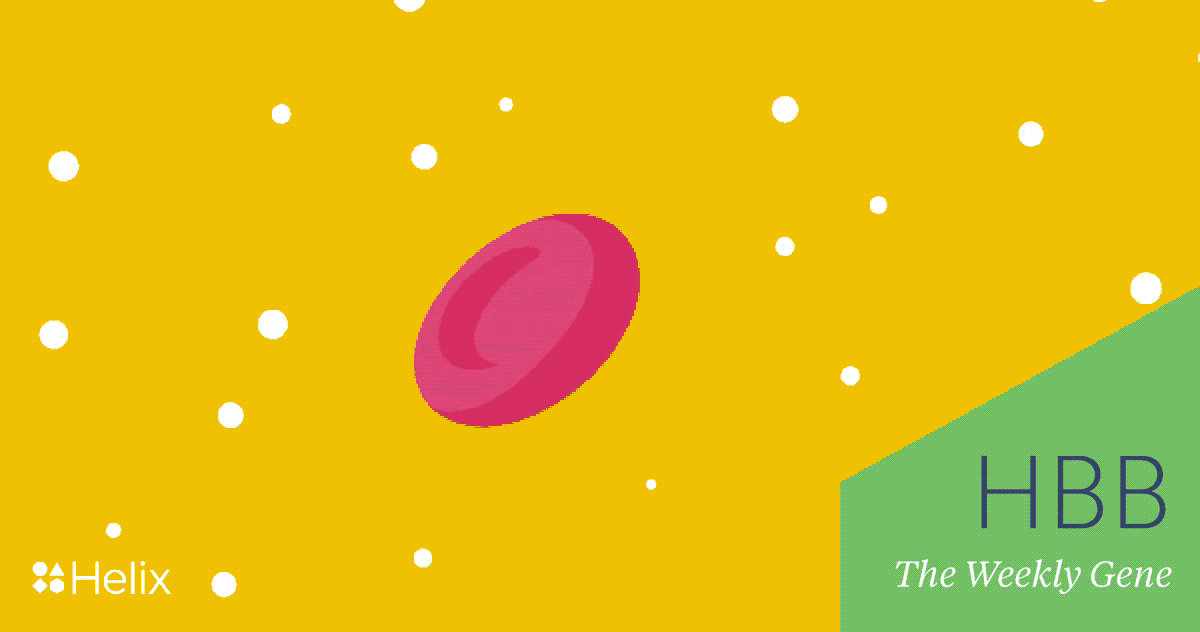
It is in the the microscopic depths of a leaf where our molecular journey begins. Formed from the fusion of carbon dioxide and water, oxygen gas ventures into the turbulence of Earth’s atmosphere where many fates await. Through chance, it may find itself being snatched from the sky and sent into the depths of our lungs.
This is where oxygen’s adventure really starts to get interesting. Being a highly vascularized organ, oxygen is easily able to diffuse from the lungs into the bloodstream. But blood is thicker than air, and it’s hard to pass freely through this dense liquid medium. As luck would have it, humans have evolved an elaborate method for carrying oxygen molecules through the blood. Iron and protein coordinate together to transport oxygen throughout the body, delivering it to every cell in the body. There, oxygen’s long journey will come to an end. To reach this final destination, however, we need a protein-metal complex that escorts oxygen through the blood. This is where the HBB gene comes into play.
Every cell requires oxygen
It’s estimated that the average adult human takes between 20 and 30 breaths per minute1,2. With each one, oxygen floods the lungs and diffuses into the surrounding blood vessels. When we think of blood, the most common image is that of red blood cells (RBCs). But blood is more than these cells that give blood its color—it’s a mixture of plasma (water, salt, and various proteins) and numerous cell types, including RBCs. As we mentioned, blood—the water component of it, specifically—poses a hurdle to oxygen which can’t easily diffuse through it. This is a major problem for the cells of your body, because every cell requires oxygen.
To overcome this barrier, our bodies build a metal protein complex known as hemoglobin. Like blood, hemoglobin describes a collection of smaller subunits3,4. In this case, the hemoglobin complex is built inside RBCs using four individual proteins. Each subunit has its own unique properties, but what’s important to know here is that they all carry an iron molecule which gives them the ability to interact with oxygen. Due to its chemical properties, iron can grab oxygen from the blood, hold it for a while, and let go of it elsewhere. This quality is critical to sustaining life: we have to be able to take oxygen from the lungs and distribute it to other parts of the body.
Various microenvironmental factors can determine when and where the iron takes up or releases oxygen4. Consider your muscles during exercise; they need a lot of oxygen to facilitate extended energy output. During exercise, the temperature of your muscles will increase and the muscle cell microenvironment will become more acidic due to lactic acid production. As it happens, increased temperatures and an acidic environment both cause iron to let go of oxygen4. Functionally, this means that as red blood cells pass through muscles, several factors are working to encourage the release of oxygen from iron in the hemoglobin.
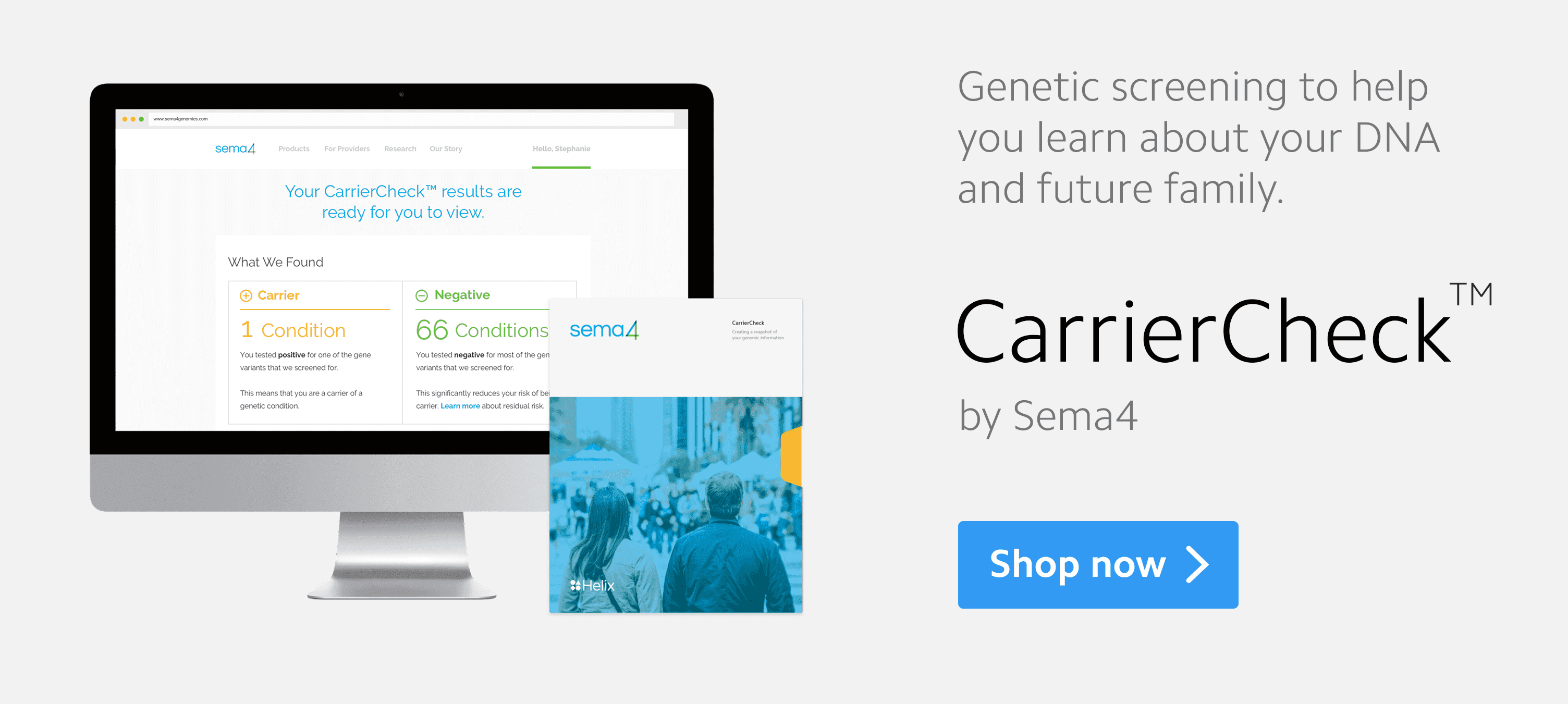
Malaria and sickle cell disease
Researchers have found that, on average, people who inherit one copy of the sickle cell disease variant are more resistant to malaria infection.
In places with a high prevalence of malaria, there tend to be more individuals heterozygous for sickle cell anemia (meaning they carry one copy of the variant). This is likely because having one copy of the variant provides an advantage, and researchers hypothesize the advantage is a resistance to malaria infection6,7.
The majority of hemoglobin in adult humans is comprised of two alpha subunits, and two beta subunits. The beta subunit is coded for by the HBB gene, which has been well-studied due to its role in sickle cell disease. This disease is characterized by a variant in the HBB gene which causes the hemoglobin beta gene to be built slightly differently. The change in this subunit causes the hemoglobin complex to change in shape, which ultimately affects the shape of the RBC. Typically, RBCs are round and disk-shaped. In sickle cell disease, however, they are crescent-shaped and resemble a sickle, hence the name5.
In order for blood to effectively deliver oxygen to our body, it must be able to flow freely through the blood vessels. Because of their shape, the RBCs affected by sickle cell disease have problems passing smoothly through vessels and are likely to clump together in various locations. When this happens, it can prevent blood flow to portions of the body that are downstream of the clot, effectively suffocating those cells, and could lead to painful blood vessel rupture. Aside from causing disruptions to blood flow, sickled RBCs have a shorter life span when compared to normal ones (sickle cells last about 10-20 days, whereas regular RBCs last 80-90 days). The short life of these RBCs can lead to anemia-like symptoms.
Sickle cell disease is the most common blood disorder in the United States, present in nearly 100,000 people. Thanks to several decades of research, we have a much greater understanding of how variants in the HBB gene lead to sickle cell disease and are better able to treat it5. Advances in technology have given us the ability to identify people with this disease very early in life—even before they’re born. In fact, using DNA sequencing, it’s possible to detect whether you have just one copy of the sickle cell variant. Sickle cell anemia only develops when a person inherits two copies of the disease associated variant; however, people who inherit a single copy are at an increased risk of having children with the disease. Genetic counseling and numerous other resources are available to help people better understand the implications of having a sickle cell variant and what it may mean for their family.
It’s clear that HBB is critical to oxygen transport, and critical to human life itself. Research into this gene, and others like it, has taught us about human disease and about our more basic molecular needs. What will we learn from our DNA next?
1“Your Lungs and Exercise.” Breathe, vol. 12, no. 1, 2016, pp. 97–100., doi:10.1183/20734735.elf121.
2Badawy, Jack, et al. “Is Everyone Really Breathing 20 Times a Minute? Assessing Epidemiology and Variation in Recorded Respiratory Rate in Hospitalised Adults.” BMJ Quality & Safety, vol. 26, no. 10, 2017, pp. 832–836., doi:10.1136/bmjqs-2017-006671.
3Billett HH. “Hemoglobin and Hematocrit.” Clinical Methods: The History, Physical, and Laboratory Examinations. 3rd edition. Boston: Butterworths; 1990. Chapter 151. Available from: https://www.ncbi.nlm.nih.gov/books/NBK259/
4Pittman RN. “Regulation of Tissue Oxygenation.” Morgan & Claypool Life Sciences; 2011. Chapter 4, Oxygen Transport. Available from: https://www.ncbi.nlm.nih.gov/books/NBK54103/
5Bender MA. “Sickle Cell Disease.” GeneReviews® [Internet]; [Updated August 17 2017]. Seattle (WA): University of Washington, Seattle; 1993-2018. Available from: https://www.ncbi.nlm.nih.gov/sites/books/NBK1377/
6Eridani, Sandro. “Sickle Cell Protection from Malaria.” Hematology Reports 3.3 (2011): e24. PMC. Web. 29 Mar. 2018.
7Taylor, Steve M., Carla Cerami, and Rick M. Fairhurst. “Hemoglobinopathies: Slicing the Gordian Knot of Plasmodium Falciparum Malaria Pathogenesis.” Ed. Chetan E. Chitnis. PLoS Pathogens 9.5 (2013): e1003327. PMC. Web. 29 Mar. 2018.