Genetics & Sleep: How Does DNA Affect Your Sleep?
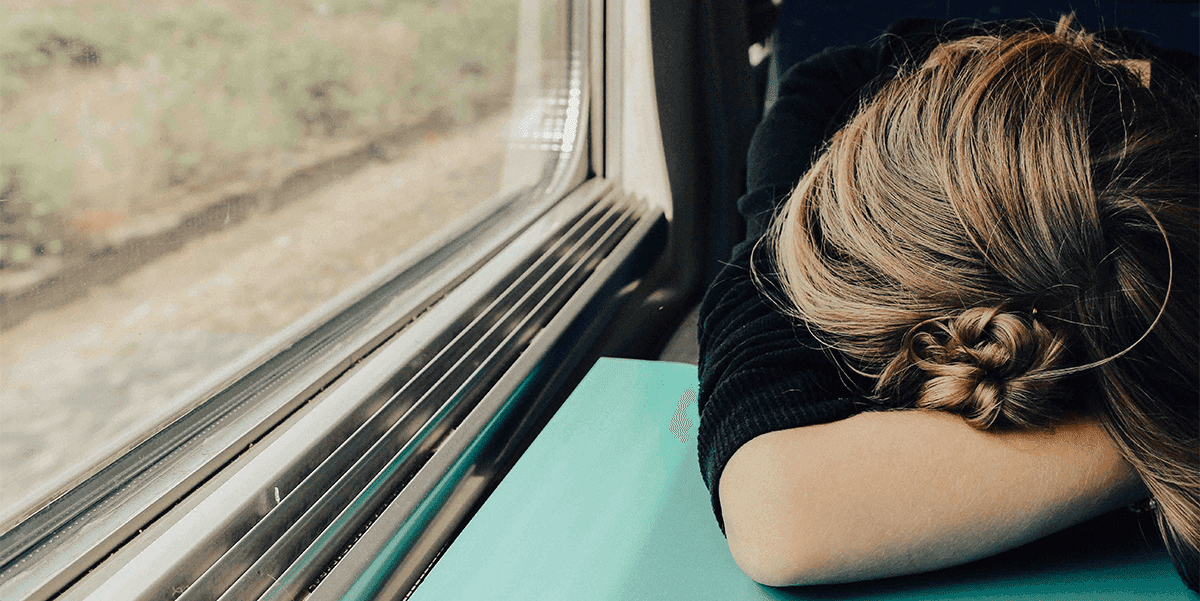
Some people just seem to be able to roll out of bed at sunrise and jump right into the day. Others are slower to wake up, but are able to be productive well into the night. Why do our sleeping habits vary so much from person to person? Scientists have asked this same question, and their research shows that DNA is part of the reason.
To say the least, sleep is a popular activity. We need it to live, and we spend about a third of our lives doing it1. We’re not alone, either—sleep has been described (in some form or another) in many different animals including birds, dolphins, flies, worms, lizards, snakes, and fish 2. Researchers believe that sleep evolved millions of years ago to help animals conserve energy, improve mental cognition, or simply pass the time between periods of feeding2.
Sleep varies from species to species. Consider the difference between dolphins and humans. Dolphins engage in a form of sleep where the two halves of their brain alternate between a sleep-like state and a more alert, awake state. Dolphins don’t have an REM cycle (the stage of sleep where we dream), possibly because REM sleep is often accompanied by muscle relaxation—which would be bad for an animal that is perpetually swimming2. In contrast, humans typically have a solid block of time where our bodies become temporarily paralyzed and the majority of our brain goes through cycles of REM and non-REM (NREM) sleep2,3. It may not be surprising that humans and dolphins sleep differently, but even among people, there’s wide variation in sleep patterns.
“Early birds” have a natural tendency to wake up earlier than most people, but are also prone to feeling sleepy earlier in the day. In contrast, the so-called “night owls” prefer to go to bed later and wake up later. Scientists studying these traits have found that the DNA we inherit may affect which category we fall into4-6.
Studies both large and small have aimed to explain how a person’s genetics might influence their sleep habits. Researchers have found that whether a person is likely to wake up early or later is partially determined by genes involved in setting their circadian rhythm—their internal clock.
Nearly every cell in the human body has its own rhythm that controls when (and to what extent) some parts of the DNA will be used. These individual rhythms are synchronized by signals sent from part of the brain known as the suprachiasmatic nucleus (SCN). Together with input from environmental factors like food, light, and temperature, the SCN helps our body know when to wake up and when to go to sleep. Critical to this process are genes like CLOCK, CRY, PER, CK1ẟ/ϵ, and DEC2, which collectively build and maintain a cell’s circadian rhythm3,4,6.
Variants—heritable changes in the DNA—that are found in these genes affect sleep to different degrees. For example, some variants in the PER2 gene have been found to increase a person’s likelihood of having Familial Advanced Sleep Phase Syndrome. People with this condition exhibit an extreme shift in their sleep cycle, resulting in a drive to fall asleep between 1:00 am and 3:00 am, and wake up between 6:00 pm and 8:00 pm4,6. But there are also less severe effects related to variants in the circadian rhythm genes. Variants in the CLOCK gene have been associated with a shift of only half an hour in sleep duration7. This highlights the fact that there’s a wide spectrum of ways that DNA can potentially influence a person’s sleep.
The Genetics of Nightmares
DNA can influence more than how long you sleep or when you go to bed. Some research also suggests that genetics may predispose people to experiencing vivid nightmares, sleep paralysis, and sleepwalking9.
An emerging area of focus for researchers relates to sleep and the immune system. The link between them is somewhat intuitive because most people are familiar with the sluggish, tired feeling that comes with illness. It’s not clear what that link is or why exactly its there, but genetic studies have found evidence that variants in the DNA affecting our immune system may also lead to changes in our sleep habits4. Good examples of this are changes in the HLA genes, genes that help our body produce antibodies to fight infections. Variants in these genes have been associated with the occurrence of narcolepsy with cataplexy, which is when an individual experiences excessive daytime sleepiness, disrupted sleep at night, and moments of temporary paralysis that’re often brought on by sudden, strong emotions4,8. Research has found that many people with narcolepsy have altered levels of a signaling protein known as orexin which helps stabilize a person in the wakefulness state8. Importantly, many people with these variants never develop narcolepsy, suggesting to researchers that narcolepsy may be the result of both environmental and genetic factors.
After decades of research, it’s clear that sleep is an important and complex activity that’s influenced by the foods we eat and drink, the environments we live in, and—to some extent—our DNA. With much left to learn, we look forward to discovering more about the latest sleep science for a long time to come.
Why Do Humans Get Tired?
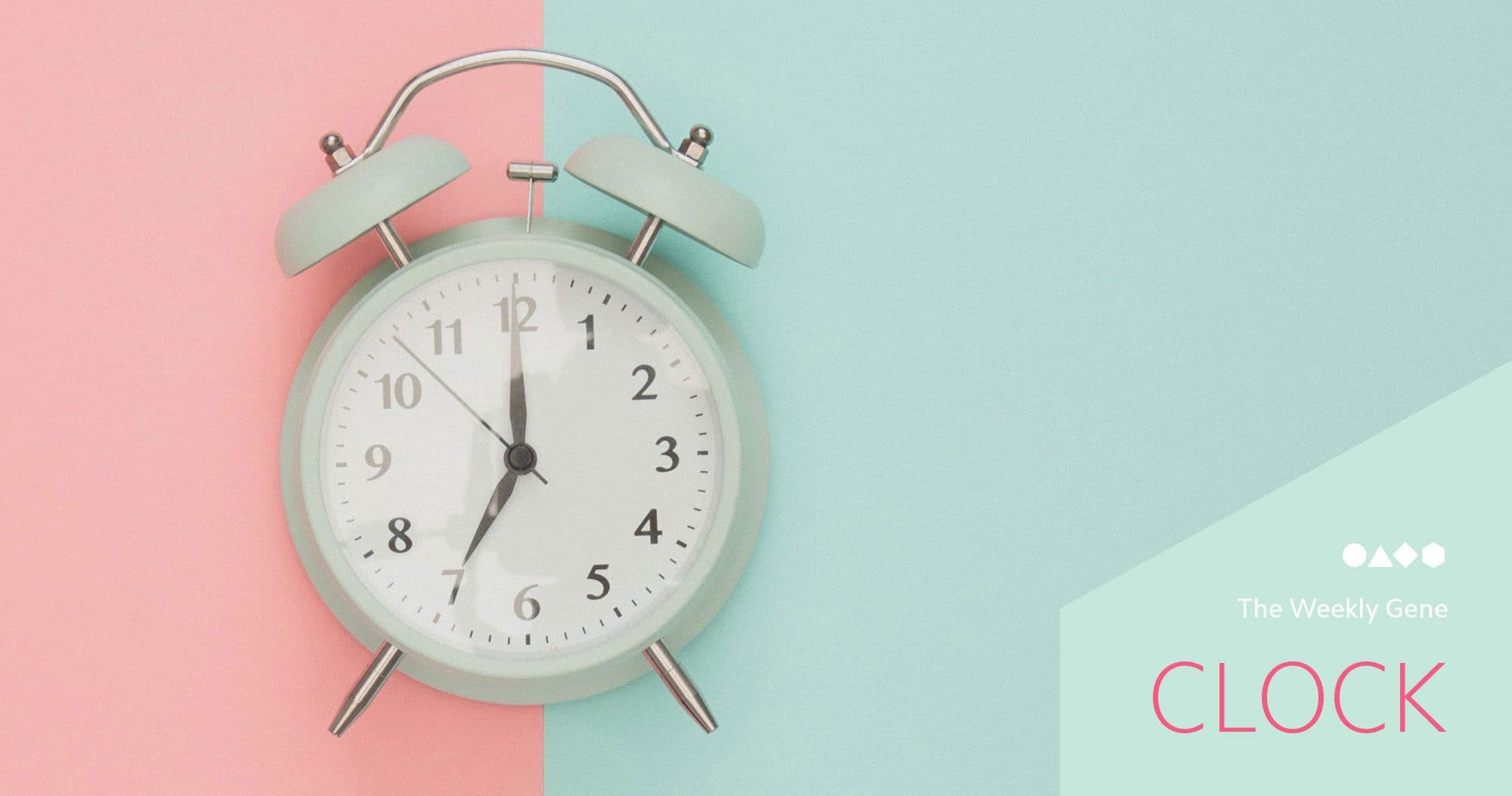
Anyone who has traveled across time zones and suffered from jet lag is likely familiar with the biological clock—also known as the circadian clock. This internal rhythm helps your body wake up, eat, and go to sleep in regular intervals. Exactly what sets your circadian clock is unknown, but it appears to involve a mixture of environmental cues (light, food, temperature) and genetics. In the same way that a mechanical clock has many pieces working together to tell time, your DNA has numerous genes working together to keep your body functioning with a predictable rhythmicity. One of the more influential genes in this genetic clock is the aptly named CLOCK gene.
More than 20 years ago, Dr. Martha Vitaterna identified the circadian locomotor out-put cycles kaput gene—otherwise known as CLOCK1. Early research in mice showed that this gene had a critical role to play in defining an animal’s biological clock. Both mice and humans show a repeating, wave-like rhythm where the peaks of the wave represent the point in a day where we are most awake. The distance between wave peaks is approximately 24 hours—meaning the time when you are the most awake one day is about 24 hours away from the time when you’ll be wide awake the next day10,11. Dr. Vitaterna and many others have shown that this 24 hour rhythm is partly controlled by the CLOCK genes.
Humans Dream More
Though we spend a third of our lives asleep, we actually sleep less than other primates. Humans typically sleep about 6.5 hours on average; compare that to orangutans, which sleep about 8-9 hours on average. Those pale in comparison to the three-striped night monkey, which sleeps nearly 15 hours a day! Despite sleeping less, humans spend more time in a rapid eye movement (REM) state, which is when dreaming occurs. So we may sleep less—but we appear to maximize our time for dreaming11.
At a molecular level, the circadian clock works through a negative feedback loop. At the start of the day, light stimulates the brain causing a cascade of hormones and electrical signaling throughout the body10,11. This cascade helps trigger the activation of the CLOCK gene and its protein product (also called the CLOCK protein). The CLOCK protein acts as a master regulator that can control the activity of other genes. As it builds up, it begins to activate numerous genes involved in increasing our wakefulness. But one of the genes targeted by the CLOCK protein, known as CRY, negatively regulates the CLOCK gene. As the CRY gene becomes more and more activated, it begins to turn off the CLOCK gene. When the CLOCK gene is turned off, it can no longer activate the CRY gene resulting in decreased activation of both. In total, this process takes about 24 hours to complete a full cycle10,11.
In summary:
- Light stimulates activation of the CLOCK gene and production of the CLOCK protein
- CLOCK protein levels rise over time
- CRY gene becomes activated as CLOCK levels begin to rise
- CRY gene begins to turn off the CLOCK gene and cease CLOCK protein production
- CLOCK protein levels decrease, resulting in decreased activation of the CRY gene
- The process repeats itself
It’s worth noting that there are numerous other genes involved in this feedback cycle that follow a similar regulatory loop. Evidence indicates that variants in the CLOCK gene can affect this cycle and result in changes to an organism’s sleep duration, quality of sleep, and the number of hours in one cycle. For example, in mice, it was shown that variants in the CLOCK gene could reduce an animal’s sleep time by as much as 2 hours. To a lesser extent, some studies have also shown that humans with a variant in the CLOCK gene sleep about a half hour longer than those without it. Research into how variants in the CLOCK gene affects sleep is still ongoing, but these early studies offer some interesting avenues for future investigation10,11.
Coffee, stress, light exposure, all of these can—and in many cases do—affect how tired people feel10-12. But research into genes like CLOCK suggest that some part of our sleep preference is influenced by our genetics. Fully understanding the genetics behind sleep will take more research, but thanks to advances in DNA sequencing technology, we’re closer than ever!
A Good CRY Goes a Long Way for Humans and Bees
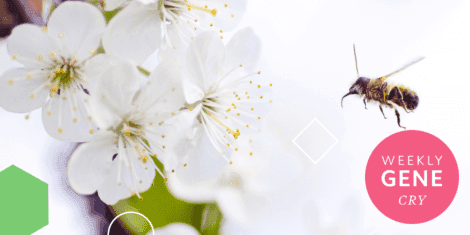
It’s like clockwork. Every day, at the same time, forager bees set out to collect nectar that will later be used to feed the hive. Their mission is not an aimless search, but rather a targeted foray guided by a mixture of sunlight and DNA. Rhythmic behavior can be found throughout the animal kingdom: bees forage, butterflies migrate, and humans sleep. Amazingly, one type of ancient gene is found in all of these animals, and it’s responsible for helping each of us keep track of time. They are known as the cryptochrome genes.
Cryptochrome genes (more commonly referred to as CRY genes) are ancient—meaning they’ve been around in some form or another for millions of years13. Early forms of life had to protect themselves from the damaging effects of light. We don’t often think about it, but light has the potential to break DNA, which can be a big problem for our cells. To combat this, cells evolved a new type of gene whose protein product could sense light and use it as a cue to start scanning the DNA for breakpoints that need repairing. Over time, this gene evolved into many different genes that have various light-related jobs. Some of those genes are now known as CRY genes13.
CRY genes make up a central part of the biological rhythm—also known as the circadian clock—in many different organisms. Humans have two different CRY genes (CRY1 and CRY2). Together, they help cells in our body progress through a 24-hour cycle. In a nutshell, our daily rhythm of waking up, eating, being active, and falling asleep follows a regular pattern. That pattern is influenced by many factors including light, food, temperature, and our genetics. Genes like the CLOCK and CRY genes help our bodies maintain this rhythm in the same way that gears in a clock help it tick away with regularity13,14.
The same is true for other organisms as well, including honey bees and butterflies13. Honey bees are an extremely social species (also known as eusocial) who participate in socially determined activities. One of these activities is when forager bees leave the hive in search of food. Researchers have found that bees can be trained to search for food at specific times and in specific intervals with a remarkable level of adaptivity15-17. While studying this behavior, researchers have also found that genes involved in maintaining a circadian rhythm—including a version of the Cry gene that’s similar to ours—are important timekeeping tools for bees. Studies suggest that circadian genes help align social behaviors across the hundreds of workers in a hive, some of which may be spread out over wide distances13,15-17.
Part of this social alignment involves the sun. To understand this, it helps to look at monarch butterflies. These insects participate in a seasonal migration from northern territories down towards Mexico each autumn13. This regular migration relies on a butterfly’s ability to sense sunlight intensities at different times of day. This is where the circadian clock is helpful. Once the change in daylight lines up appropriately with the circadian clock, butterflies can be cued to migrate13,18.
CRY vs Cry?
Did you notice a formatting change in our text? It was no accident, capitalization of gene names can tell you a lot about the gene. Here, the full capitalization of CRY indicates that it’s referring to a human gene. The other format, Cry, tells you it’s referring to a gene in one of multiple animal groups that includes insects.
In each of these animals, Cry proteins are important elements of their internal clock. It’s important to note that the CRY genes are not exactly the same in humans as they are in bees or butterflies, but they’re very similar13. These differences may impact how the gene is regulated and ultimately affect different species’ circadian rhythms differently.
We can see this within humans, too. Some people have small changes in their CRY genes that affect their sleep habits14,19. One such change happens in the CRY1 gene and is associated with a condition known as Familial Delayed Sleep Phase Syndrome, where a person tends to fall asleep later than the societal average (sometimes by hours)19. This is an example of a dramatic shift in sleep that can result from genetic changes, but there are also more subtle ones that we’ve reviewed here.
Ultimately, the CRY genes provide a great example of an ancient gene that’s been passed down for millions of years. These genes have evolved to serve the specific needs of animals (and plants). Whether in bees, butterflies, or humans, the CRY genes help maintain life’s many rhythms.
Daylight Savings Time: Turn Back Your Clocks and Tune into Your Genes
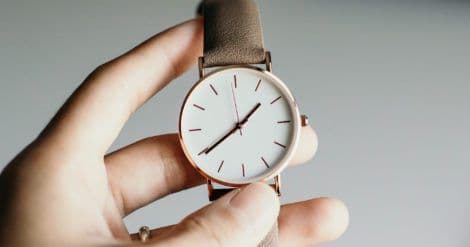
As Daylight Saving Time once again comes to an end, we must roll the clocks back, sacrificing that precious hour of evening daylight. It’s a simple process—adjust the clock forward one hour in the spring, and back an hour in the fall—but changing your internal clock is another story. Humans have evolved a complex system that keeps our biology synchronized with the daily cycles of sunrise and sunset. Everyone has a personal internal rhythm which may be affected by Daylight Saving differently. Whether you’re an early riser or a night owl, how your body adapts to this change might be affected by ancient remnants of Neanderthal DNA.
The concept of Daylight Saving started in the early 1900s as a mechanism to conserve energy, shifting our day to maximize natural light during working hours in order to reduce usage of electric light. A common assumption is that DST will affect someone’s sleep patterns only on the day when clocks are changed. But researchers have found that sleep can be affected for much longer, sometimes altering a person’s sleep patterns for weeks20. Interestingly, these effects appear to be partially dependent on a person’s chronotype.
The term chronotype refers to a person’s natural sleep patterns. Some people prefer to stay up later than most (night owls), while others are early to bed and early to rise. Where you fall on this spectrum is referred to as your chronotype. The factors determining your chronotype are not fully understood, but researchers have found that both environmental cues and genetics can play a role. How you respond to the start of Daylight Saving (in the spring) versus how you respond to its end (in the fall) can be partially due to your chronotype. Research shows that people who prefer staying up late are more affected by the spring transition, and less so by the fall transition21.
Those who prefer waking up early are inversely affected. Even though there are different chronotypes, most people have a similar rhythmicity that dictates how long they sleep. This rhythmic system is referred to as the circadian rhythm, a term derived from the Latin words circa and dies which roughly translate to “around a day.”
The circadian rhythm is a natural regulatory mechanism embedded in all living creatures which helps manage biological processes. Deep within the brain there is a structure known as the Suprachiasmatic Nucleus (SCN) where our circadian rhythm is controlled.
Each day, light enters our eye and stimulates a relay of signals from the retina back to the SCN. From the SCN, signals are sent throughout the body, which helps organs and cells tune their own internal clocks and ensure that body systems are synchronized. This complex system is finely tuned and allows our activities (waking up, eating, going to bed) to be synchronized with the daily cycles of sunlight. In fact, a recent study indicates that our eyes are incredibly sensitive to light, so much so that we can detect the presence of a single photon22. The evolution of such a finely honed tool is one of many adaptations we’ve accumulated throughout time. Being able to detect changes in our environment and adapt our internal clock has allowed us to explore new areas of the world and eventually establish a human presence across the globe.
Some of these adaptive abilities may have come from non-human ancestors. More than 30,000 years ago, Homo sapiens coexisted with a similar hominid known as Homo neanderthalensis, or more commonly Neanderthals. These close relatives began to breed with Homo sapiens which led to hybrid genomes composed of human and Neanderthal DNA. Over time, most of the Neanderthal DNA has been lost. Some Neanderthal DNA has persisted, though, and may account for up to 2% of the DNA in people of Eurasian descent. The function of this Neanderthal DNA is still being investigated, but new research sheds some light on a common feature—much of the persistent Neanderthal DNA affecting human traits is associated with light23.
Some of these traits include skin color, hair color, and even a person’s chronotype. In this study, compelling data suggests that a person’s preference for early wakefulness or late bedtimes might be influenced by regions of DNA that trace back to Neanderthal ancestors. Like modern humans, Neanderthals may have had a spectrum in these types of traits as well. Further analysis of the data allowed the researchers to speculate on why this DNA has continued to be a part of our genome—they suggest it may be an adaptation to geographical locations where sunlight exposure is different from the equator.
Daylight Saving Time is an old custom, but not as old as our biological clock. The effect of this time change is small, but based on your chronotype—and, indirectly, on your ancestral DNA—you may experience it differently than others.
Citations
- “Brain Basics: Understanding Sleep.” National Institute of Neurological Disorders and Stroke, U.S. Department of Health and Human Services, www.ninds.nih.gov/Disorders/Patient-Caregiver-Education/Understanding-Sleep.
- Joiner, William J. “Unraveling the Evolutionary Determinants of Sleep.” Current biology : CB 26.20 (2016): R1073–R1087. PMC. Web. 11 Sept. 2018.
- Wright, Kenneth P., Christopher A. Lowry, and Monique K. LeBourgeois. “Circadian and Wakefulness-Sleep Modulation of Cognition in Humans.” Frontiers in Molecular Neuroscience 5 (2012): 50. PMC. Web. 10 Sept. 2018.
- Sehgal, Amita, and Emmanuel Mignot. “Genetics of Sleep and Sleep Disorders.” Cell 146.2 (2011): 194–207. PMC. Web. 10 Sept. 2018.
- Kalmbach, David A. et al. “Genetic Basis of Chronotype in Humans: Insights From Three Landmark GWAS.” Sleep 40.2 (2017): zsw048. PMC. Web. 11 Sept. 2018.
- Andreani, Tomas S. et al. “Genetics of Circadian Rhythms.” Sleep medicine clinics 10.4 (2015): 413–421. PMC. Web. 10 Sept. 2018.
- Landgraf, Dominic, et al. “Clock Genes and Sleep.” SpringerLink, Springer, Dordrecht, 11 Aug. 2011, link.springer.com/article/10.1007/s00424-011-1003-9.
- Leonard, C S, and J P Kukkonen. “Orexin/hypocretin Receptor Signalling: A Functional Perspective.” British Journal of Pharmacology 171.2 (2014): 294–313. PMC. Web. 12 Sept. 2018.
- Hublin, Christer, et al. “Parasomnias: Co-Occurrence and Genetics.” Psychiatric Genetics, vol. 11, no. 2, 2001, pp. 65–70., doi:10.1097/00041444-200106000-00002.
- Landgraf, Dominic, et al. “Clock Genes and Sleep.” SpringerLink, Springer, Dordrecht, 11 Aug. 2011, link.springer.com/article/10.1007/s00424-011-1003-9.
- Nunn, Charles L., David R. Samson, and Andrew D. Krystal. “Shining Evolutionary Light on Human Sleep and Sleep Disorders.” Evolution, Medicine, and Public Health 2016.1 (2016): 227–243. PMC. Web. 12 June 2018.
- Buysse, Daniel J. “Sleep Health: Can We Define It? Does It Matter?” Sleep 37.1 (2014): 9–17. PMC. Web. 12 June 2018.
- Chaves, Inês, et al. “The Cryptochromes: Blue Light Photoreceptors in Plants and Animals.” Annual Review of Plant Biology, vol. 62, no. 1, Feb. 2011, pp. 335–364., doi:10.1146/annurev-arplant-042110-103759.
- Jagannath, Aarti et al. “The genetics of circadian rhythms, sleep and health” Human molecular genetics vol. 26,R2 (2017): R128-R138.
- Rubin, Elad B et al. “Molecular and phylogenetic analyses reveal mammalian-like clockwork in the honey bee (Apis mellifera) and shed new light on the molecular evolution of the circadian clock” Genome research vol. 16,11 (2006): 1352-65.
- Lehmann, Marina et al. “The early bee catches the flower – circadian rhythmicity influences learning performance in honey bees, Apis mellifera” Behavioral ecology and sociobiology vol. 65,2 (2010): 205-215.
- Bloch, Guy. “The Social Clock of the Honeybee.” Journal of Biological Rhythms, vol. 25, no. 5, 2010, pp. 307–317., doi:10.1177/0748730410380149.
- Reppert, S. M. “The Ancestral Circadian Clock of Monarch Butterflies: Role in Time-Compensated Sun Compass Orientation.” Cold Spring Harbor Symposia on Quantitative Biology, vol. 72, no. 1, 2007, pp. 113–118., doi:10.1101/sqb.2007.72.056.
- Patke, Alina et al. “Mutation of the Human Circadian Clock Gene CRY1 in Familial Delayed Sleep Phase Disorder” Cell vol. 169,2 (2017): 203-215.e13.
- Harrison Y. “The impact of daylight saving time on sleep and related behaviours.” Sleep Medicine Reviews, W.B. Saunders, 7 Mar. 2013,
- Lahti, Tuuli A et al. “Transitions into and out of Daylight Saving Time Compromise Sleep and the Rest-Activity Cycles.” BMC Physiology 8 (2008): 3. PMC. Web. 3 Nov. 2017.
- Tinsley, Jonathan N. et al. “Direct Detection of a Single Photon by Humans.” Nature Communications 7 (2016): 12172. PMC. Web. 3 Nov. 2017.
- Dannemann, Michael, and Janet Kelso. “The Contribution of Neanderthals to Phenotypic Variation in Modern Humans.” The American Journal of Human Genetics, vol. 101, no. 4, 5 Oct. 2017, pp. 578–589., doi:10.1016/j.ajhg.2017.09.010.