What causes color-blindness?
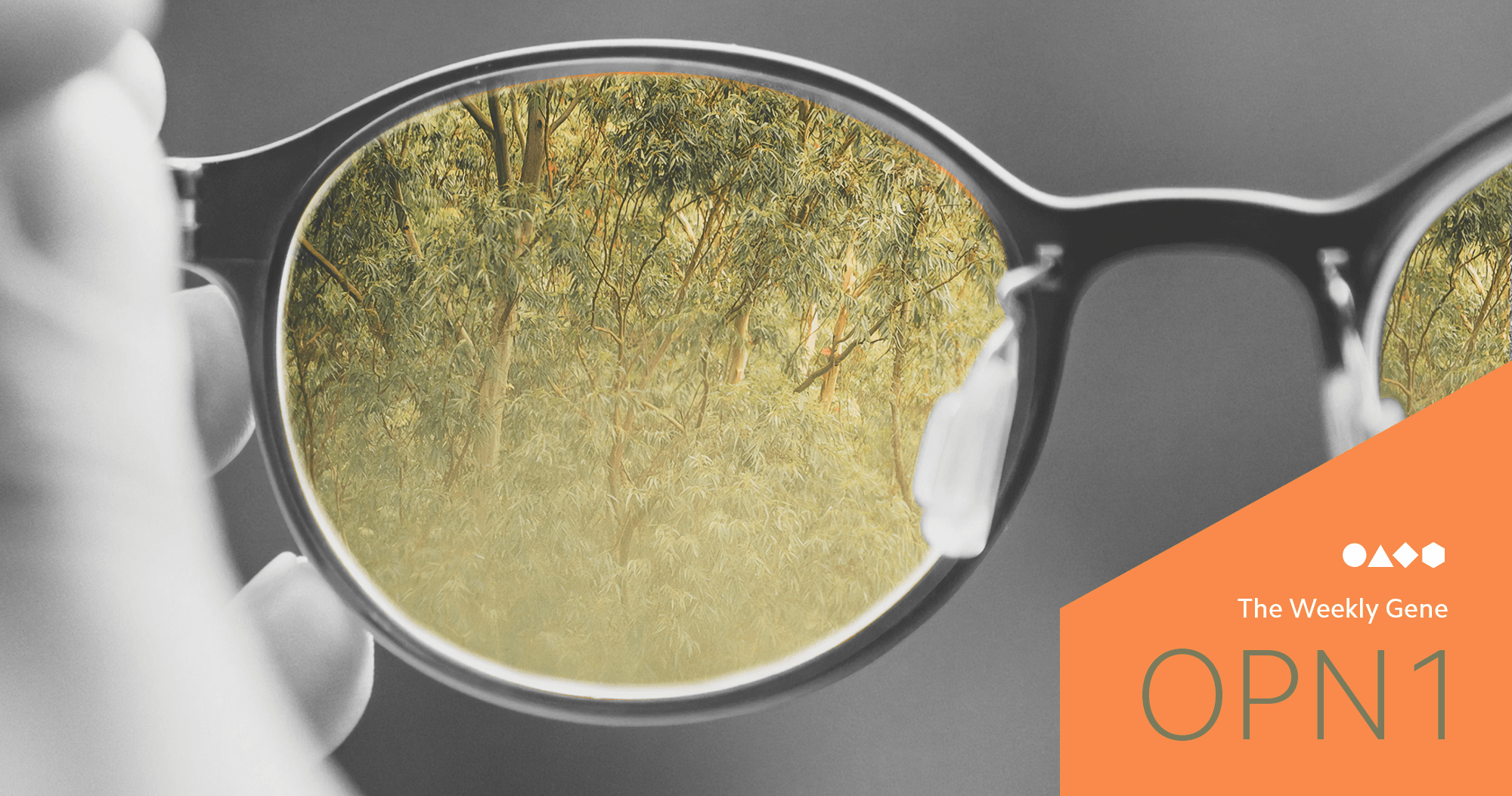
300 nanometers—that’s nearly 3,000 times smaller than a pin head—is all that separates melancholy shades of blue from the vibrancy of red. Within the tiny range of just 300 nanometers exists a whole spectrum of information in the form of visible light. Using the full range of these colors, flowers can attract or repel insects, animals can hide from predators and prey, and pyrotechnic specialists can dazzle audiences. But in order to tap into this treasure trove of color, our body has to have a key. That key is known as OPN1.
Whether it’s glacier blue, ocean green, or crimson red, all colors are a form of light which differ in the amount of energy they carry. In essence, light is just energy that’s moving through space as a wave. How much energy light has can be represented by the distance between wave peaks (also known as the wavelength). For instance, when a light has high amounts of energy, it will generate waves quickly and the distance between the waves will be short. In contrast, when there is lower energy in light, the waves will emanate at a slower pace and thus the distance between waves will be larger. Humans can detect only a small range of wavelengths between about 400 nanometers and 700 nanometers, which gives us colors that range from violet to red respectively. Though this range is incomprehensibly small, it can be used to decipher the delicate shades of blue, yellow, and green in a field of flowers or to identify edible foods from toxic ones.
Variants in opsins can cause color blindness
To use this information, though, we have to be able to capture it. That’s where the opsins come into play. Opsin genes produce light sensitive proteins that go by the same name (a common practice in genetics). When light comes into contact with opsin proteins, it transfers some of its energy to the protein (in the same way that a ball hitting a wall will transfer its energy to the wall). If there is enough energy in the light, it will cause the opsin protein to move and release its grip on retinal—another molecule that’s bound to the opsin. By hitting the opsin with enough energy, light is able to liberate retinal which goes on to trigger a relay of signals that ultimately cause our brain to recognize that light is present1. This is the basic mechanism for how we see.
For a primitive animal trying to navigate the world, the ability to detect light is good, but being able to sense the difference between high-energy wavelengths and low-energy wavelengths is even better. The ability to distinguish between different colors came about when animals evolved different versions of the opsin proteins with different sensitivity to light. These opsins are in the opsin-1 gene (OPN1) family.
OPN1 gave us the ability to see color by producing three different types of opsin2. The OPN1-LW gene produces an opsin that only releases its hold on the retinal molecule when hit by low-energy, long-wavelength light (such as red or yellow light). When this happens, our brain recognizes that something yellow or red is present. To see other shades of yellow and green, the OPN1-MW gene comes into play. The opsin produced by this gene is stimulated by the medium-wavelength colors (some yellows and green). You can probably guess how we see blues and violets: the OPN-SW gene produces an opsin which only responds to high-energy, short-wavelength colors.
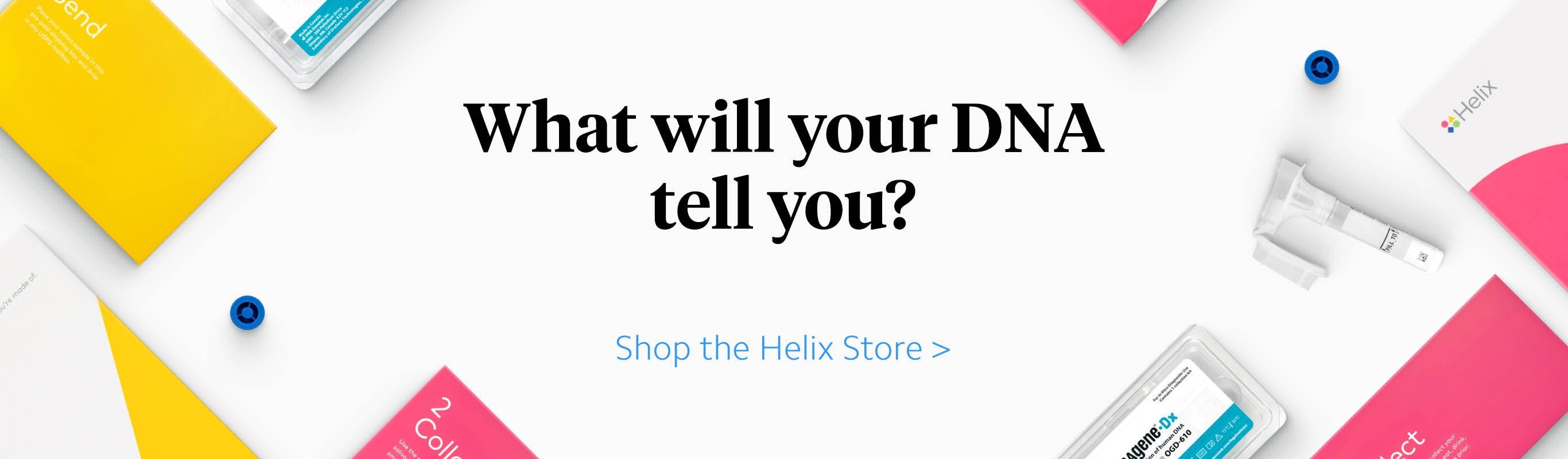
X-linked recessive
This term describes a certain pattern of inheritance. Here, the term “X-linked” means the trait is inherited through the X chromosome. Females inherit two copies of the X chromosome, which means they have two chances to inherit fully functional opsin genes. Because red-green colorblindness is a recessive trait, a female would have to inherit two copies of the altered opsin genes to be color-blind. Males, on the other hand, only inherit one X chromosome and are thus more likely to be red-green color-blind because they only have to inherit one version of the altered gene.
Over time, genetic research has helped us see how color vision can vary among species and among people. For people of European descent, about 1 in every 12 males and 1 out of every 200 females is born with a diminished ability to differentiate between red and green colors (also known as red-green color blindness)2. This can happen for a number of reasons, including changes in the DNA coding for the OPN1-LW gene that prevent its opsin from working. This condition is more common in males because the OPN1-LW and OPN1-MW genes are carried on the X chromosome and are inherited in an X-linked recessive fashion. Other, less common forms of color blindness result from changes in the OPN1-SW gene (blue cone monochromacy) or alterations to the OPN1-MW DNA sequence (blue-yellow color blindness)2. In addition to genetic reasons, there are some rare occasions where color blindness can be caused by non-genetic factors.
Light is a powerful source of energy and is rich with information. Thanks to the OPN1 genes, we can use that light to enjoy the breathtaking array of colors that are all around us.
1Terakita, Akihisa. “The Opsins.” Genome Biology 6.3 (2005): 213. PMC. Web. 08 July. 2018.
2Neitz, Jay, and Maureen Neitz. “The Genetics of Normal and Defective Color Vision.” Vision research 51.7 (2011): 633–651. PMC. Web. 11 July 2018.