Oxygen can be wild—your DNA helps tame it
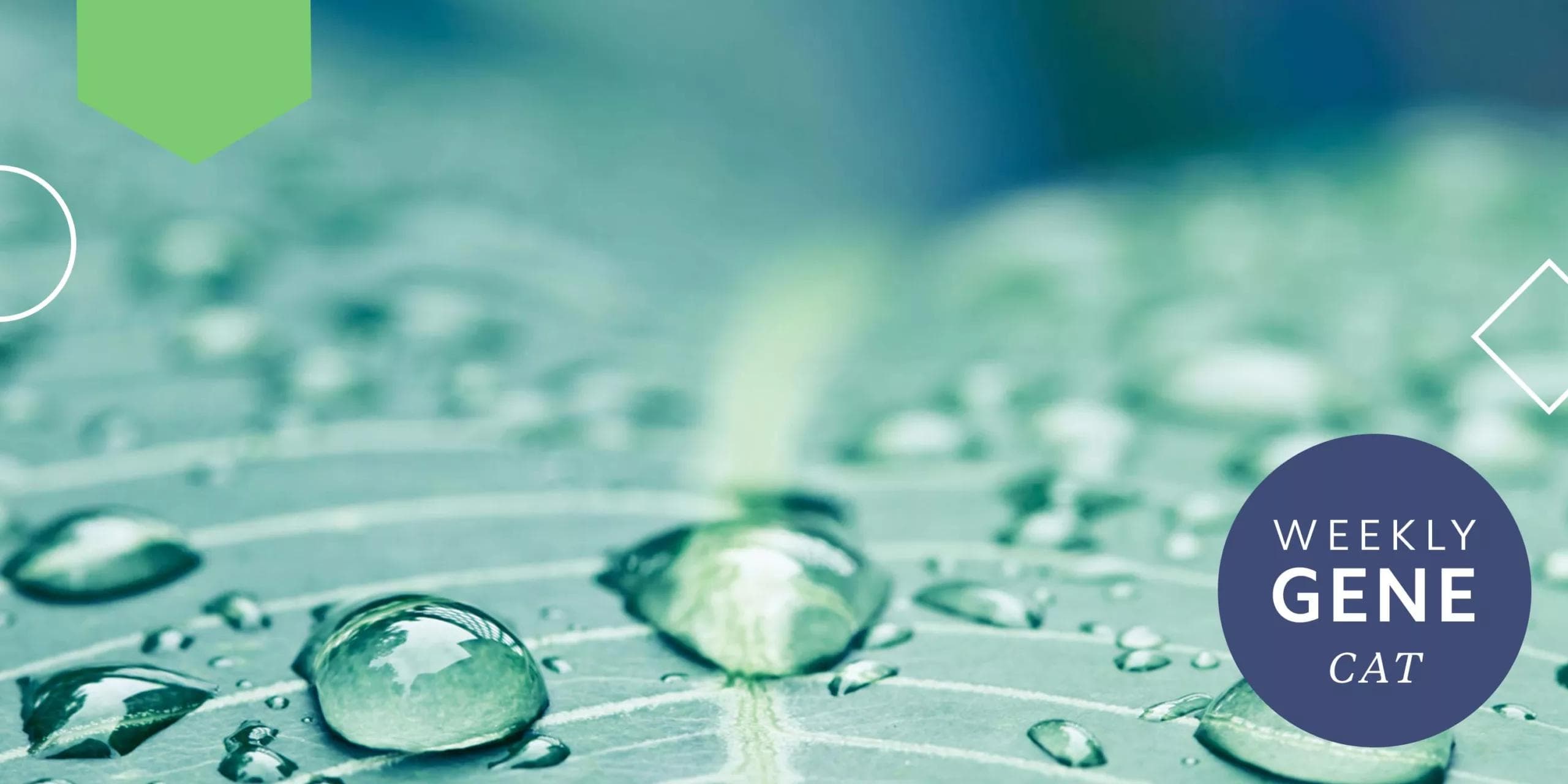
Training for a marathon and powerlifting require two very different routines, but you’re likely to come across one line of advice no matter what your goal is: Breathe! That’s because our muscles need oxygen to produce energy—the same energy that helps you lift weights and run long distances. But while oxygen is vital, it also has destructive potential. Highly unstable forms of oxygen called oxygen radicals are generated during exercise and have the power to destroy proteins, wreak havoc on DNA molecules, and even kill the muscle cells they’re in. To prevent this from happening, your body relies on protective proteins, one of which is simply known as catalase.
The oxygen we breathe is made from two oxygen molecules tightly bound to one another—represented as O2. Once in our lungs, the oxygen diffuses into our bloodstream where its can be distributed throughout the body. If you’ve ever taken a biology course, you may know what happens next: the O2 molecule is sent to the mitochondria—popularly referred to as the “powerhouse” of the cell—where the two oxygen molecules are split apart, and then converted into water and carbon dioxide. Cells do this because it helps them generate energy that’s used elsewhere in the cell.
What’s important to know is that splitting of the O2 molecule is where things can go wrong. Once separated, the oxygen molecules form what’s known as reactive oxygen species (ROS) which are extremely unstable—meaning they’re quick to latch onto nearby proteins, fats, and other chemicals. When this happens, it can alter (and sometimes destroy) the chemical structure of whatever it latches onto. Most of the time, our cells are able to keep control of ROS and prevent them from escaping the mitochondria. But occasionally, some ROS do break loose, and that’s where antioxidant systems come into play.
While oxygen is vital, it also has destructive potential
One of those antioxidant systems involves catalase, a specialized protein whose job is to neutralize specific types of ROS (H2O2) before they can do any harm. When a cell detects that ROS levels are rising, it will respond by turning on the CAT gene which then tells the cell how to make more of the catalase protein. This is how catalase protects our muscles during exercise1,2.
In order to meet the demands of weight lifting, running, hiking, and other forms of exercise, our muscles have to strike a balance between generating and neutralizing ROS1. In some situations, ROS can actually serve an important role that helps normal cell function. In muscle cells, ROS can promote the release of calcium stores that then flood the cell and cause it to contract. Even though ROS are generated to help the muscle contract, some leak out from the mitochondria. The combined effect of this is an increase in the amount of potentially damaging ROS during exercise, which needs to be carefully regulated1. To prevent unwanted effects from ROS, catalase (along with other similar proteins) helps defend our muscle cells from damage and keeps them healthy1,2. But for some people, a small change in the DNA sequence coding for CAT can decrease their ability to neutralize ROS.
Genetic studies have found that a single base change in the CAT gene can diminish a person’s ability to make catalase3-6. Exactly what effect this has on a person’s muscles during exercise is unclear, but it’s believed that lower levels of catalase may lead to increased muscle damage during strenuous workouts that would require longer periods of rest. Some studies suggest that it may be possible to counterbalance this effect by eating fruits and vegetables that are rich in antioxidants (like vitamins C and E)6. Research into how this change in the CAT gene affects athleticism and whether a person’s diet can overcome this impact is a topic of ongoing research. Notably, some findings indicate that a person’s ethnicity and gender may modify the effect of this variant, which highlights the fact that regulation of ROS is a complex process that involves many factors beyond catalase6.
1He, Feng et al. “Redox Mechanism of Reactive Oxygen Species in Exercise.” Frontiers in Physiology 7 (2016): 486. PMC. Web. 29 Aug. 2018.
2Nadif, Rachel et al. “Association of CAT Polymorphisms with Catalase Activity and Exposure to Environmental Oxidative Stimuli.” Free Radical Research39.12 (2005): 1345–1350. PMC. Web. 29 Aug. 2018.
3Saify, Khyber, Iraj Saadat, and Mostafa Saadat. “Influence of A-21T and C-262T Genetic Polymorphisms at the Promoter Region of the Catalase (CAT) on Gene Expression.” Environmental Health and Preventive Medicine 21.5 (2016): 382–386. PMC. Web. 29 Aug. 2018.
4Kopp, Tine Iskov et al. “Association between Single Nucleotide Polymorphisms in the Antioxidant Genes CAT, GR and SOD1, Erythrocyte Enzyme Activities, Dietary and Life Style Factors and Breast Cancer Risk in a Danish, Prospective Cohort Study.” Oncotarget 8.38 (2017): 62984–62997. PMC. Web. 29 Aug. 2018.
5Bastaki, Maria, et al. “Genotype Activity Relationship for Mn-Superoxide Dismutase, Glutathione Peroxidase 1 and Catalase in Humans.” Pharmacogenetics and Genomics, vol. 16, no. 4, 2006, pp. 279–286., doi:10.1097/01.fpc.0000199498.08725.9c.
6Ahn, J. “Associations between Catalase Phenotype and Genotype: Modification by Epidemiologic Factors.” Cancer Epidemiology Biomarkers & Prevention, vol. 15, no. 6, Jan. 2006, pp. 1217–1222., doi:10.1158/1055-9965.epi-06-0104.